My
research focuses on the following aspects of theoretical
condensed matter physics
Properties of Mesoscopic and Nanoscale Electron Devices
Kinetics of ferromagnetic
metal nanoparticles (model)
Devices for Quantum
Information Processing
Spin dynamics in double quantum
dots (
poster)
Noise of Electric
Current in Mesoscopic Systems
Influence of inelastic
scattering on shot noise
Current noise due to external time-dependent perturbations
Non-Linear phenomena
in disordered two-dimensional electron systems
Microwave-induced
magneto-oscillations in 2DEG
Zero-resistance state of irradiated 2DEG
Non-linear current-voltage characteristic of 2DEG
Signatures of the
Kondo effect in electron transport
Transport properties of metal
wires with magnetic impurities
Interaction effects between quantum dots in the Kondo
regime
Superconductivity
Proximity effect in disordered
and ballistic mesoscopic systems
Charge and Heat transport through superconductors close to the
phase transition
Non-equilibrium and Inelastic processes in superconductors
Classical-to-Quantum
Crossover
Brief description of my
research activities
I specialize in
theoretical condensed matter physics. I have been
developing novel theoretical techniques to describe front-line
experiments in quantum electronics and related fields. My
primary interest is understanding the kinetics of
out-of-equilibrium quantum systems. In such systems, the electron
behavior is determined not only by the low-energy electron quantum
states, but also by various relaxation mechanisms, which usually
originate from the many-particle interaction of electrons with
each other or with phonons. Kinetic theory on which I focus
analyzes the current experiments and paves the way for further
development of quantum electronics. Examples of quantum
electron systems are semiconducting quantum dots, ultra-small
metal grains and wires, and two-dimensional electron
heterostructures.
Kinetics of quantum systems is a fast growing field, which is
important for understanding and characterization of electron
properties of new materials and for development of nanoscale
electronics. The latter promises such fundamental
breakthroughs as a realization of solid state devices for quantum
information technologies.
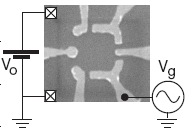
In
collaboration with other researchers, I have succeeded in
presenting an analytical description of several puzzling
phenomena discovered experimentally. Particularly, together with
I. Aleiner and V. Ambegaokar, I have described the transport
through a constrained metallic region, known as a quantum dot,
subject to time-dependent perturbations. By working beyond
the adiabatic approximation, we demonstrated that at high
frequencies the generated direct current can be attributed to a
new shape of the electron distribution function in the dot. My
further work on the analysis of such system led to
characterization of other measurable parameters of the system and
a quantitative comparison of the theory with experiments done at
Harvard University (Picture on the left: microphoto of the device
used in Harvard experiments).
I. Aleiner and I have also constructed a kinetic theory of
two-dimensional electron systems (2DES) placed in a magnetic field
in the presence of high-frequency electric fields. Later,
together with I. Dmitriev, A. Mirlin and D. Polykov, we applied
this theory to the description of the experiments on 2DES subject
to high-frequency radiation. We demonstrated that the large
amplitude of magneto-oscillations in the experimentally relevant
regime is arising from a specific non-monotonic form of the
distribution function of electrons in 2DES due to the
high-frequency fields. I plan to consider an arbitrary shape of
the impurity scattering potential and to investigate the
non-linear current in response to a constant electric field
investigated experimentally very recently. Another important
direction of my research would be to re-apply this theory to
an atomic sheet of carbon, a new type of 2DES called graphene.
In the above cases, the external field was considered classically.
To describe the interaction effects with quantized electromagnetic
fields, such as fields containing small number of
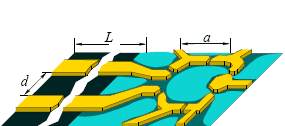
photons in its modes or fields describing electron-electron
interactions, one has to modify the developed techniques. Such
modification was carried out in my recent paper with A. D. Stone,
where we calculated electric current through a quantum dot coupled
to quantized electromagnetic field (A schematic picture of the
device is shown on the right). In the near future, I plan to
further develop the theory of electron transport through quantum
systems and consider how different types of interaction influence
transport characteristics of these systems. In addition to the
Coulomb interaction, I will analyze the interaction between
electrons in the Cooper channel that leads to the appearance of
superconductivity. Of particular importance is to calculate the
charge and heat conductances and current noise of small
superconducting devices close to the superconducting phase
transition because these devices are currently the subject a broad
experimental investigation. Such devices are important for
practical realizations of ultra-sensitive detectors of various
spectra of electromagnetic radiation. They also address such
fundamental questions as the thermodynamic and quantum phase
transitions in metal-superconductor systems.